The Oxygen-Iodine Laser (OIL) operates on the spin-orbit transition of atomic iodine I(2P1/2) → I(2P3/2) + hv, inverted through energy transfer from singlet oxygen O2(a1Δ) via the process
O(a1Δ) + I(2Ρ3/2)
→ O2(X3Σ) + I(2P1/2).
This laser has a number of excellent features for
industrial applications particularly in the dismantling of nuclear reactors. Potential applications include directed
energy weapons, heavy materials processing etc.
One of the urgent problems to be solved for the development of high-power pulsed and cw OILs is the construction of high-pressure O2(a1Δ) generators. In the case of pulsed OILs, this will provide an increase in the specific energy extraction from the active medium volume. For cw OILs with the supersonic flow of the active medium, an increase in the initial oxygen pressure leads to the increase in the Mach number by preserving the high density of O2(a1Δ) in the resonator and also simplifies a system for waste gas exhaust to the atmosphere. OILs based on a high-pressure Singlet-Oxygen Generator (SOG) do not require water vapour traps.
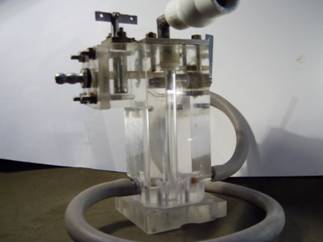
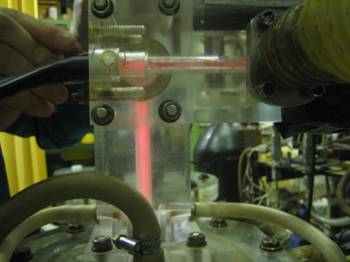
Photo 1. High pressure a jet-droplet type SOG. The left photo is the general view of SOG. The right photo shows the SOG in operation.
Experiments were carried out on a setup whose schematic diagram is shown in Fig. 2. Singlet oxygen was generated in a counterflow jet singlet oxygen generator which had a reaction zone cross section of 8x1.2 cm2. In all experiments, the chlorine flow rate was 22±0.3 mmole/s. BHP solution was prepared using 3 liters of 37% hydrogen peroxide solution and 2.3 liters of KOH solution at a concentration 14.5 mole/L. The solution temperature was maintained at -8…-14°С.
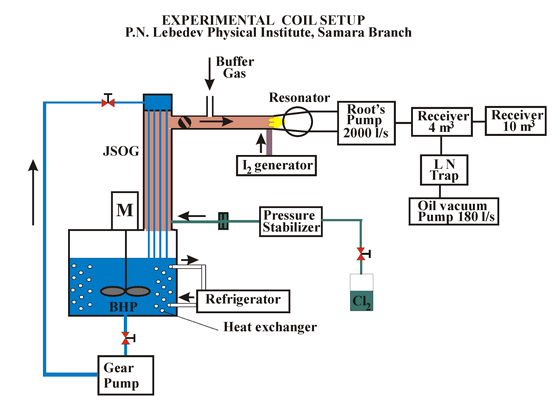
The SOG and the resonator were connected through an organic glass channel of length 7.5 cm and cross section 10×1.2 cm2. Molecular iodine vapour was introduced into the oxygen flow by a rake-type injector consisting of 16 vertical copper tubes with ten holes of diameter 0.5 mm drilled on both sides of each tube at right angles to the oxygen flow. Primary buffer gas was injected into the oxygen flow before the I2 injector. We used a 64-cm long stable resonator with overall mirrors transmittance T=1.3%. The diameter 4.5 cm of the mirrors determined the downstream length of active zone. The optical axis of the resonator was on distance of 5 cm downwards on a stream from a plane of input of iodine with secondary buffer gas. The gain length was 10 cm. Fig. 3 represents the photo of SOG attached to the resonator.
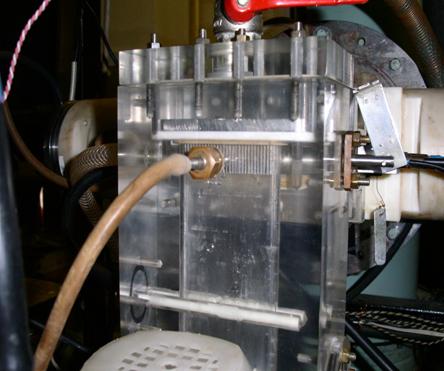
Fig. 3. A view of the jet-type SOG attached to the resonator.
It is shown that the substitution of the buffer gas N2 by CO2 does not cause any significant variation in the dependence of the output power on the degree of dilution of the active medium. The maximum power was 581 W for the flow rate of molecular chlorine 22 mmole/s that corresponds to a chemical efficiency of 29 % (Antonov I.O. et al. Apll. Phys. Lett., 2006, vol. 89, p. 051115(3), doi: 10.1063/1.2222405). The results of our research are protected by patent RU # 2390892.
COIL with external iodine atom generator. The harnessing of molecular iodine as a precursor for iodine atoms is convenient to use because in this case the external iodine generator does not needed. One way to further improve COIL performance is to avoid the loss of O2(a1Δ) molecules associated with the dissociation of I2. Estimations predicted a substantial increase in chemical efficiency, if one could inject iodine atoms instead of molecules. Many researchers from Japan, USA, Israel, Czech Republic and Russia investigated the potentialities of external production of I atoms. Kadymova and coworkers (Institute of Physics, Czech Republic) significantly contributed to develop the chemical and electric-discharge methods of iodine atom production.
We performed a straightforward comparison of two methods of iodine production – conventional, by means of I2 dissociation in the singlet oxygen flow and with iodine atoms produced externally in an electric discharge. It was found that the singlet oxygen lifetime was about 30% longer when iodine was produced from CH3I in the discharge, as compared to the conventional I2 dissociation in the singlet oxygen flow (Mikheyev P.A. and Azyazov V.N., J. Appl. Phys., 2008, vol. 104, p. 123111(6), doi: 10.1063/1.3050339). Therefore, using an atomic iodine generator in COIL can increase the inversion lifetime in the laser medium. This is important for efficient resonator designs that require an extended region of positive gain along the flow.
We demonstrated a cw COIL operation in the subsonic mode with
external production of iodine atoms in CH3I/Ar
dc glow discharge. Figure 4 shows the photo of COIL with a electric-discharge iodine atom generator. The cw COIL was powered by a centrifugal bubble SOG that was developed in
Dr. Zagidullin's lab. The outputs of a dc
glow discharge sustained in Ar/CH3I
gas mixture used in our experiments was extensively studied by Mikheyev
and coworkers (Mikheyev et al. J. Phys. D: Appl. Phys., vol. 37, p.
3202, 2004, doi:
10.1088/0022-3727/37/22/024).
We made a straightforward comparison of COIL performance for two cases – conventional, when I2 was injected in the singlet oxygen flow, and when iodine atoms produced externally together with other discharge products were injected. In the latter case nearly four times increase in output power was observed. Figure 5 represents the dependences of COIL output power on iodine concentration for two methods of I atoms production.
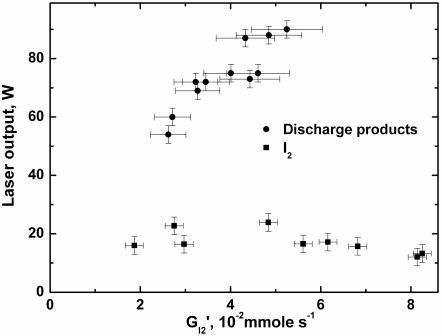
Four times increase of laser output was observed in experimental conditions unfavorable for conventional COIL due to high water content. The results prove that a substantial amount of iodine atoms had been transported to the injection point. It was shown that the products of methyl iodide dissociation have little or no adverse effect on COIL performance. To make further progress it is necessary to understand which iodine donor (CH3I, CF3I, HI, I2, etc.) and what type of electric discharge are best suited for the efficient production of I atoms. This work was supported by RFBR grant # 08-02-12035.
The
main results:
1.
Obtained a record density of electronically excited molecules
O2(a1Δ) up to 1018 cm-3 at gas temperatures close to
ambient. (Azyazov V.N. et
al. Quantum Electronics,
vol. 24, 1994, p.
120-123,
doi: 10.1070/QE1994v024n02ABEH000033).
3. Demonstrated efficient operation of COIL in subsonic and supersonic modes with CO2 buffer gas. (Antonov I.O. et al. Apll. Phys. Lett., 2006, vol. 89, p. 051115(3), doi: 10.1063/1.2222405).
4. Find the values of the similarity criteria under with the
effective power extraction from the cw OIL active medium is achieved. (Mezhenin A.V. and Azyazov V.N.,
IEEE Quantum Electron. v. 49, p. 739-746, 2013, doi: 10.1109/JQE.2013.2272097).